What is Hemolytic Uremic Syndrome (HUS) and what do I need to know about it?
Hemolytic uremic syndrome was first described in 1955, but it was not known to be secondary to Escherichia coli (E. coli) infections until 1983. HUS is now recognized as a cause of acute kidney failure in infants and young children. Adolescents and adults are also susceptible, as are the elderly, who often have severe disease and are at significant risk of death from the disease.
Most E. coli strains live harmlessly in human intestines and rarely cause disease in healthy individuals. In fact, E. coli is part of the normal ecosystem in warm-blooded animals.
How did otherwise-harmless E. coli strains become such killers? Genome sizes of E. coli can differ by a million base pairs between commensals (i.e. “normal flora”) and pathogenic variants, and this extra genetic content can contain virulence and fitness genes. Comparative genomics have shown that E. coli genomes are split between a shared, conserved set of genes, called the core genome, and a flexible gene pool. The pathogenic ability of E. coli is largely afforded by the flexible gene pool through the gain and loss of genetic material at a number of hot spots throughout the genome. It is likely that DNA from a Shiga toxin-producing bacterium known as Shigella dysenteriae type 1 was transferred by a bacteriophage (a virus that infects bacteria) to harmless E. coli bacteria, thereby providing them with the genes to produce one of the most potent toxins known to man—so potent, in fact, that the Department of Homeland Security lists Shiga toxin as a potential bioterrorist agent. Although E. coli O157:H7 is responsible for the majority of cases in the U.S., there are many additional Shiga toxin-producing E. coli strains that can cause HUS. A large outbreak in Germany and northern Europe in 2011 was caused by the E. coli O104:H4 strain; this strain contained two plasmids—one which conferred increased ability of the Shiga toxin-producing E. coli to adhere to mucosa and one which conferred antibiotic resistance.
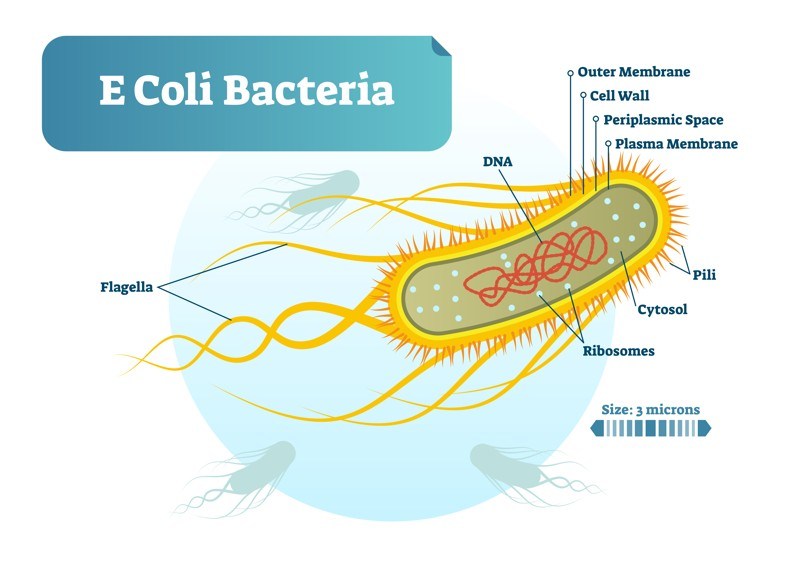
Shiga toxin-producing E. coli-associated HUS (STEC-HUS) belongs to the body of thrombotic microangiopathies, a heterogeneous group of diseases characterized by the triad of features: thrombocytopenia (low platelet count), mechanical hemolytic anemia with schistocytosis (“schistocytes” in the blood, which are damaged red blood cells), and ischemic organ damage. STEC-HUS is caused by gastrointestinal infection by a Shiga toxin-producing E. coli (and occasionally other Shiga-toxin-producing pathogens) and is also called “typical” HUS—as opposed to “atypical” HUS, which results from alternative complement pathway dysregulation, and “secondary” HUS, which is caused by various co-existing conditions.
Post-diarrheal HUS (D+ HUS) is a potentially severe, life-threatening complication that occurs in about 10-15% of those infected with E. coli O157:H7 or other Shiga toxin-producing E. coli (STEC). HUS not preceded by diarrhea is signified as “D- HUS.”
From Diarrhea to Dialysis
The chain of events leading to HUS begins with the ingestion of STEC—for example, E. coli O157:H7—in contaminated food or beverages, as a result of exposure to animals carrying the bacteria, or from person-to-person transmission. In the past, ingestion of undercooked hamburger was a common source of STEC infections; however, more recent epidemics have been associated with ingestion of contaminated food items such as apple juice, radish sprouts, sausages, strawberries, and more. While epidemics are notable, the majority of HUS cases from STEC are sporadic—without a specific source for the STEC being identified. It is noteworthy that E. coli can survive for months in the environment, potentially leading to the contamination of fresh produce.
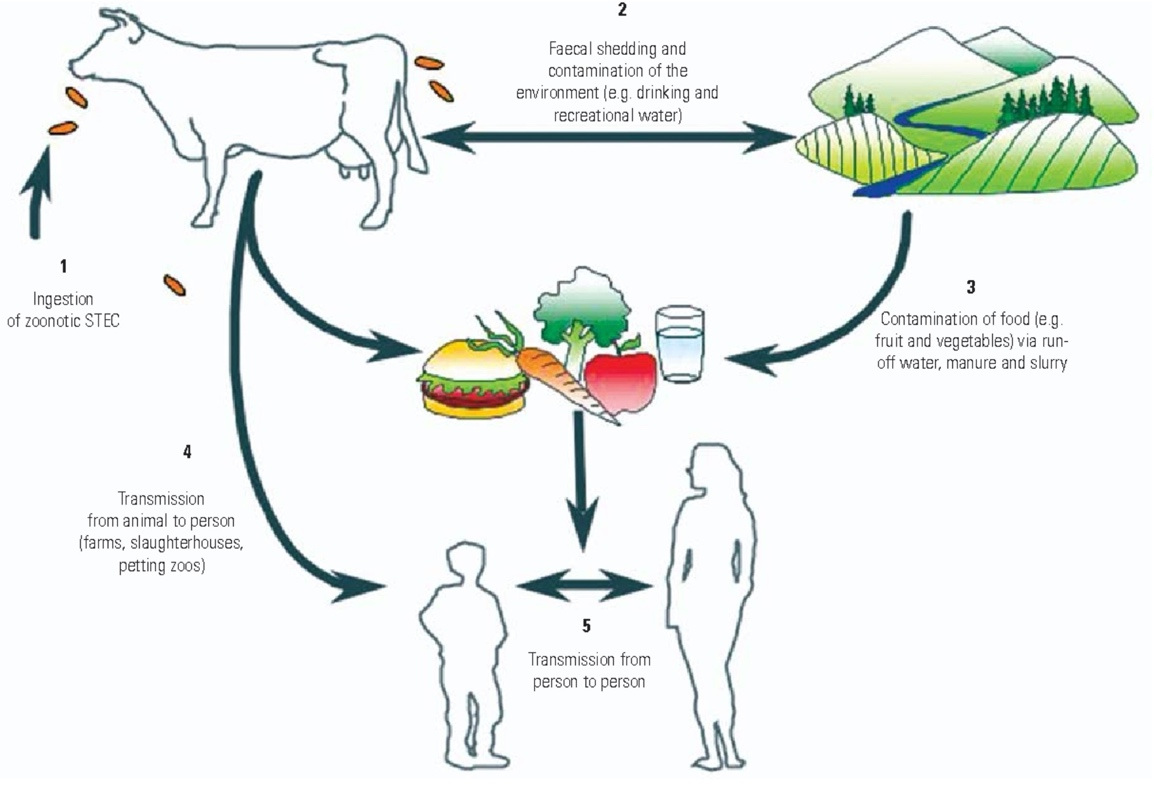
These E. coli multiply rapidly in the colon, causing colitis and diarrhea, and tightly binding to cells that line the large intestine. This snug attachment facilitates absorption of the toxin into the intestinal capillaries and into the systemic circulation, where it becomes attached to weak receptors on white blood cells, thus allowing the toxin to “ride piggyback” to the kidneys. From there, it is transferred to numerous avid (strong) Gb3 receptors that grasp and then hold on to the toxin.
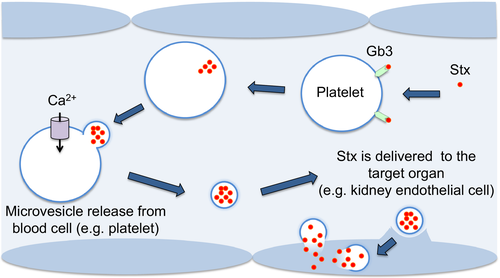
Organ injury is primarily a function of receptor location and density. The receptors are probably diversely distributed in the major body organs; this may explain why some patients develop injury in different organs—for example, the brain or pancreas. While the kidney is the organ most commonly affected in HUS, extra-renal manifestations of HUS are common. Extra-renal disease is the major cause of mortality related to HUS.
Once the Shiga toxins attach to receptors, they move into the interior of the cell (cytoplasm). There, they shut down the protein machinery, resulting in cellular injury or death. Cellular injury activates blood platelets and the “coagulation cascade,” causing the formation of clots in the very-small vessels of the kidney. These clots, in turn, result in acute kidney injury (AKI) and acute renal failure (ARF).
AKI in STEC-HUS patients ranges from asymptomatic urine sediment abnormalities to severe renal failure and end-stage renal disease. Proteinuria is usually mild and has been described in 30% of patients, combined with hematuria in 6.6% and leukocyturia (white blood cells in the urine) in 26%. Between 30% and 61% of STEC-HUS patients require renal replacement therapy (RRT; i.e. hemodialysis or peritoneal dialysis) during the course of the disease, with a mean duration of oliguria or RRT of 9-10 days. Fifteen percent of children develop hypertension.
Red blood cells are either destroyed by the Shiga toxin (hemolytic destruction) or damaged as the cells attempt to pass through partially-obstructed microvessels. Blood platelets (thrombocytes), which are required for normal blood clotting, become trapped in tiny blood clots or are damaged and destroyed by the spleen.
The kidney and brain are the organs most vulnerable to STEC-HUS, but several other organ involvements have been described and need to be considered when evaluating patients with STEC-HUS.